Toward Patient-Specific Surgical Planning Including Individualized Physiology Modeling
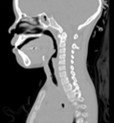
Introduction
This blog introduces a clinical application that Kitware is developing to combine physiologic modeling with patient-specific surgical planning. The project integrates a surgical planning tool for treatment of narrowing of the subglottic airway with a whole-body open-source physiology engine (extended from BioGears) that is being developed at Kitware. Combining individualized physiology simulations with patient-specific surgical training can provide clinicians with a more complete picture of the potential patient outcomes.
Clinical problem
Airway problems in pediatric patients may cause life threatening respiratory difficulties, poor growth, aspiration, delay in speech development and long-term, and debilitating illness [1]. One common upper airway problem is subglottic stenosis, or narrowing of the airway below the vocal cords. Treatment of the stenosis generally requires surgery. The surgical plan is currently developed using tests such as rhinomanometry and subjective evaluation of the CT (Computed Tomography) scans. However, in many cases, the treatment plan is informed by other factors, including clinician preference and experience or other institutional preferences. With the growing interest and drive towards precision, quantitative, and personsonalized medicine, surgical planning tools with patient-specific capabilities can play an important role in improving patient outcomes.
Methodology
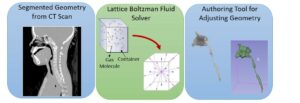
Virtual Pediatric Airway Workbench (VPAW) is a surgical planning tool for subglottic stenosis that incorporates three major components [1]. First, VPAW initiates with a CT scan of the patient and obtains a geometrical model through segmentation and surface reconstruction. Second, it employs a computational fluid dynamics (CFD) engine based on a Lattice-Boltzmann formulation- fluid is modeled as particles travelling in discrete directions as shown in Figure 1. Exchanges of momentum and energy occur during collisions between particles and the boundary. This formulation is used to compute the airflow in the nasal cavity. Finally, it also provides a real-time geometric authoring tools that allow surgeons to edit the tracheal geometry using a haptic device as part of the surgical planning.
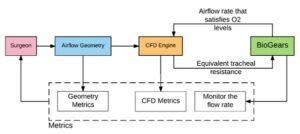
VPAW allows for patient-specific geometry to drive the characterization of the airflow in the large airways; however, it does not incorporate the relevant physiology of the individual patient. Without patient physiology, the effects of changing airflow on critical physiologic outcomes, such as respiration rate and oxygen saturation, are not part of the surgical plan. As a proof of concept the open-source physiology engine, was interfaced with VPAW [2]. The respiratory system of the physiology engine is linked to the CFD model in VPAW via the tracheal resistance in the lumped parameter model.
The respiratory rate and oxygen saturation resulting from various degrees of tracheal stenosis are shown in Figure 3. By calculating the respiration and oxygen saturation due to the stenosis, surgical planning can determine the optimal treatment for the overall physiologic health of the patient. This preliminary result was presented at SPIE Medical Imaging Conference in Orlando 11–16 Feb 2017 [2]. This not only shows a strong proof of concept, but also exposes the exciting possibilities in advancing patient-specific surgical planning factoring in individualized physiology. The majority of physiology engines, open-source in particular, only model adult physiology; therefore, pediatric modeling is a critical need for further development of patient-specific treatment and planning tools. Kitware is working on this with several clinical collaborators.
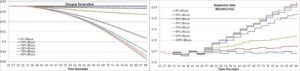
Summary
The blog provides just one example of how Kitware is developing patient-specific surgical planning and training applications that incorporate physiological modeling. To learn more about Kitware’s open source physiological modeling development efforts, please contact kitware@kitware.com.
References
[1] Quammen C., Taylor R., Krajcevski P., Mitran S., Enquobahrie A., Superfine R., Davis B., Davis S., Zdanski C., “The Virtual Pediatric Airways Workbench”, Proceedings of Medicine Meets Virtual Reality (MMVR) 22, Los Angeles, CA., April 7-9, 2016.
[2] Potter L., Arikatla S., Bray A., Webb J., Enquobahrie A., “Physiology informed virtual surgical planning: a case study with a virtual airway surgical planner and BioGears”, Proc. SPIE 10135, Medical Imaging 2017: Image-Guided Procedures, Robotic Interventions, and Modeling, 101351T (March 3, 2017); doi:10.1117/12.2252510.